Representations and neural circuits of emotional memories
Emotion has a powerful impact on memory. While memory of the mundane fades quickly, emotionally significant events often make a lasting impression. Particularly, threats possess an especially potent ability to enhance memory due to the fact remembering dangerous situations is evolutionary advantageous. Given that misjudging a threat can mean the difference between life or death, it comes as no surprise that fear of a particular stimulus can be acquired rapidly and maintained for long periods of time after only a single exposure. Importantly, the memory of a fearful event involves two different types of processing: a) encoding the specific details of the event to prevent encountering the same situation in the future, and b) extracting the general features of a fearful situation to generalize behavioral responses to similar scenarios that can be potentially dangerous. The Muzzio lab is interested in understanding how the brain codes these distinct processes at the single cell and population level and determine the mechanisms that lead to abnormal over-generalization.
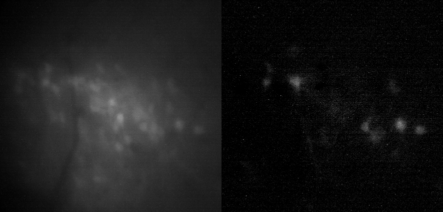
i. Subcortical encoding of emotional memories
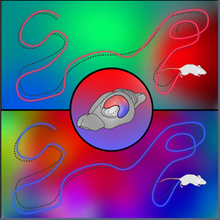
Information about context is represented in the hippocampus. Evidence in support of this role comes from the tendency of neurons in this region to be active in particular locations when an animal moves through an environment. However, hippocampal connectivity and place cell characteristics change significantly along the longitudinal (dorso-ventral) axis, suggesting that the dorsal (DH) and ventral (VH) regions may have distinct functions. The Muzzio lab has formulated and tested the alternative idea that emotional and spatial information are integrated along the longitudinal hippocampal axis and that the VH, which connects with other areas involved in emotional learning, gates the expression of generalization processes. The lab is currently studying the following questions: How is the VH representing generalized features of emotional memory encoding? What VH projections control specificity vs. generalized response patterns?
ii. Cortical encoding of emotional memories
While the role of the hippocampus in the formation of episodic memory is well established, it is also known that crosstalk between hippocampus and cortical regions leads to the formation of cortical memory traces that can be retrieved independently of the hippocampus as time passes by (a process known as systems consolidation). The lab is investigating the cortical representations associated with recent and remote memory in the medial prefrontal and auditory cortices to address the following questions: 1) How do connections between cortical regions affect recent and remote memories? 2) How do cortical networks change when memories are consolidated at recent vs. remote time points? 3) How do cortical representations represent generalized fear responses?
Impact of aging and sleep on memory
Age-related changes in sleep patterns have been linked to cognitive decline. Specifically, age is associated with increased fragmentation during sleep and wake cycles and alterations in cortical spindles, fast oscillations that play an important role in memory consolidation. The Muzzio lab has recently demonstrated that NREM sleep consolidation improves the flexibility of hippocampal representations and enhances place/object recognition memory in old animals. Moreover, successful memory correlates with increased number of spindles. These findings suggest that improvements in sleep quality may serve to ameliorate age-related cognitive decline. Current topics of interest include studying if changes in sleep quality affect replay of memories during sleep and wake periods.
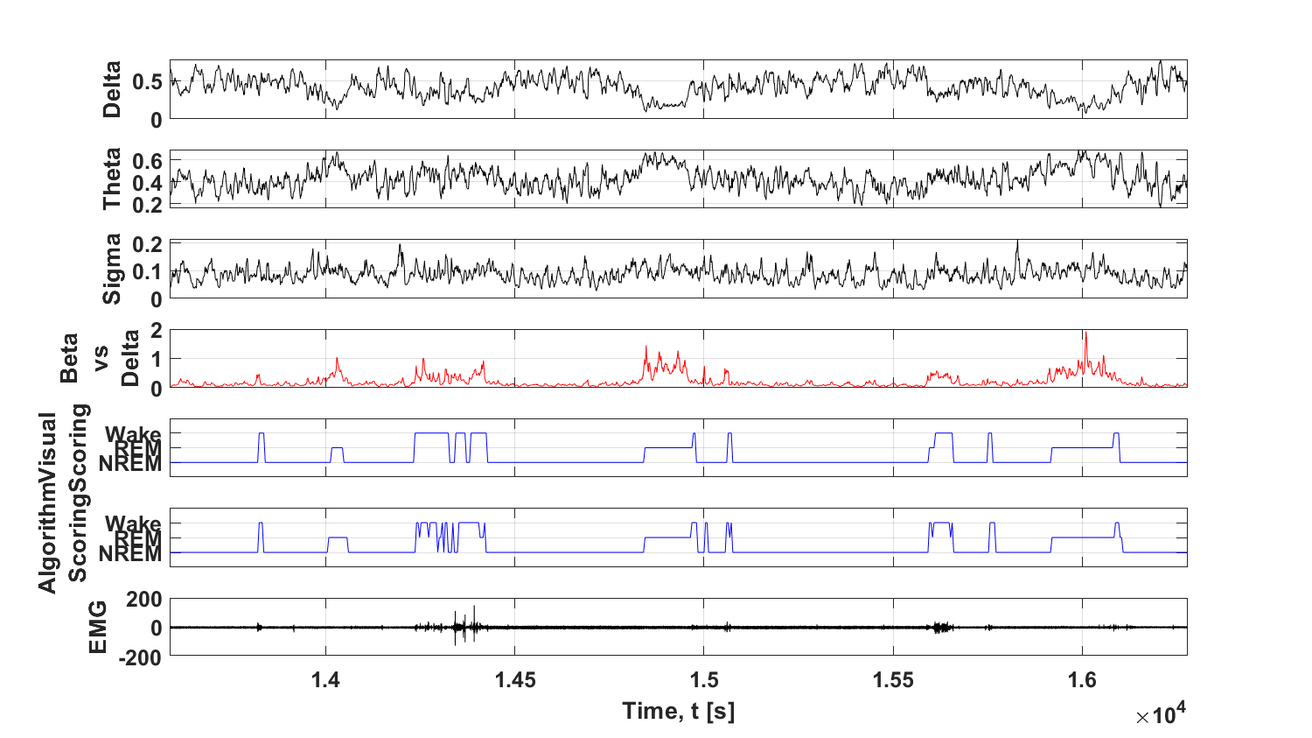
Spatial navigation
I. Brain representations of spatial reorientation
During navigation, there are numerous sensory cues that are used to determine a subject’s surroundings and facing direction, such as landmarks (e.g., objects, odors, signs, etc.) and the shape of a layout (i.e. geometric cues). Typically, this information is integrated with self-motion cues to establish a navigators’ location. However, during disorientation self-motion cues are unreliable, which forces navigators to reorient only using external cues. Although there are many external cues that can be used to regain one’s bearings, it has been shown across species that the shape of the spatial layout plays a predominant role in reorientation. This has led researchers to postulate that reorientation is driven by an allocentric (environment-centered) strategy that uses a geometric module to guide behavior. The Muzzio lab has found that the hippocampal map aligns to geometry during reorientation and this alignment serves to predict behavior. However, disoriented navigators learn to incorporate non-geometric information to reorient with experience, indicating that while there is a strong tendency to use geometry-based strategies, these representations are not impermeant. The lab is currently investigating how the brain controls the use of different reorientation strategies in order to maximize reward.
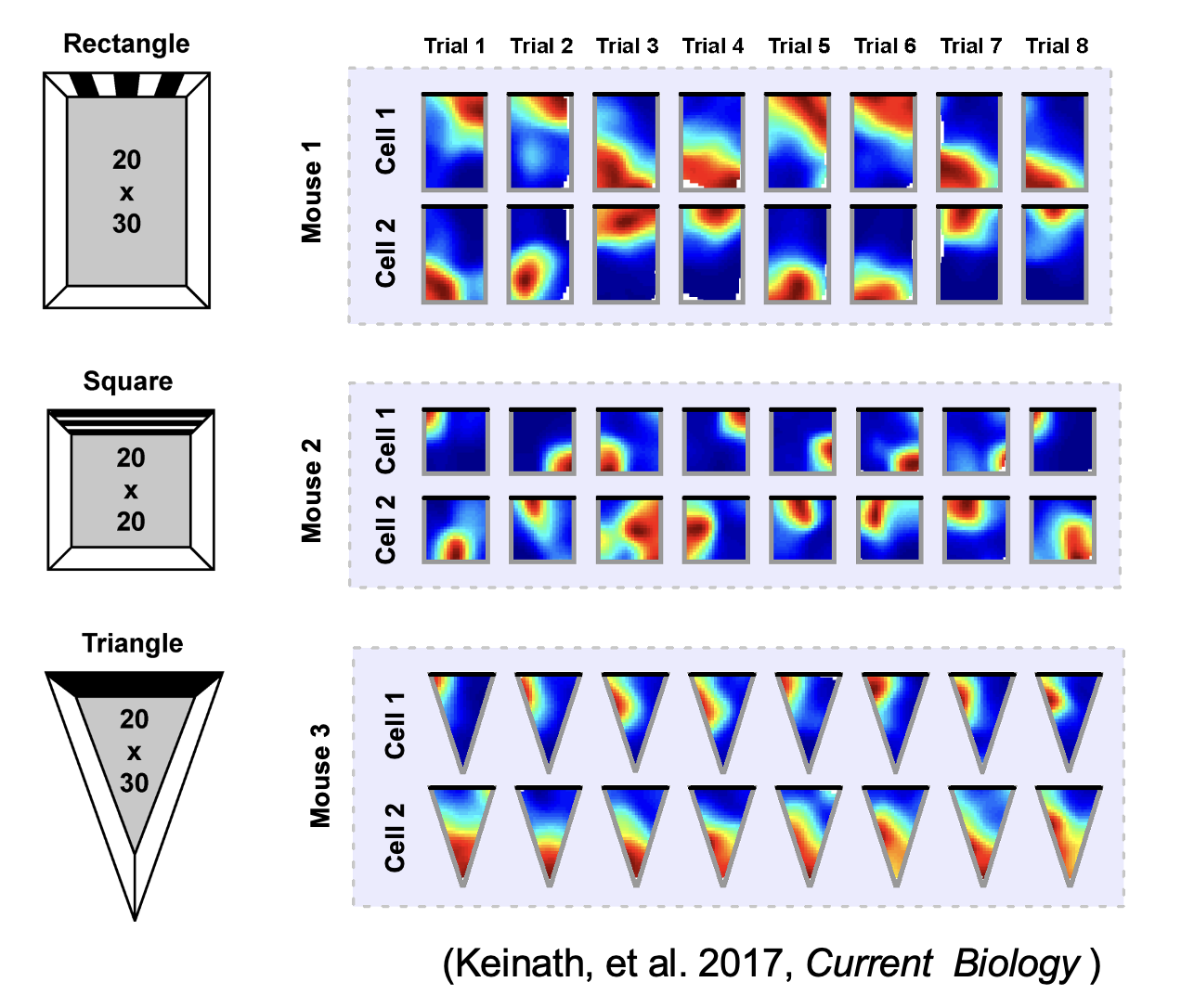
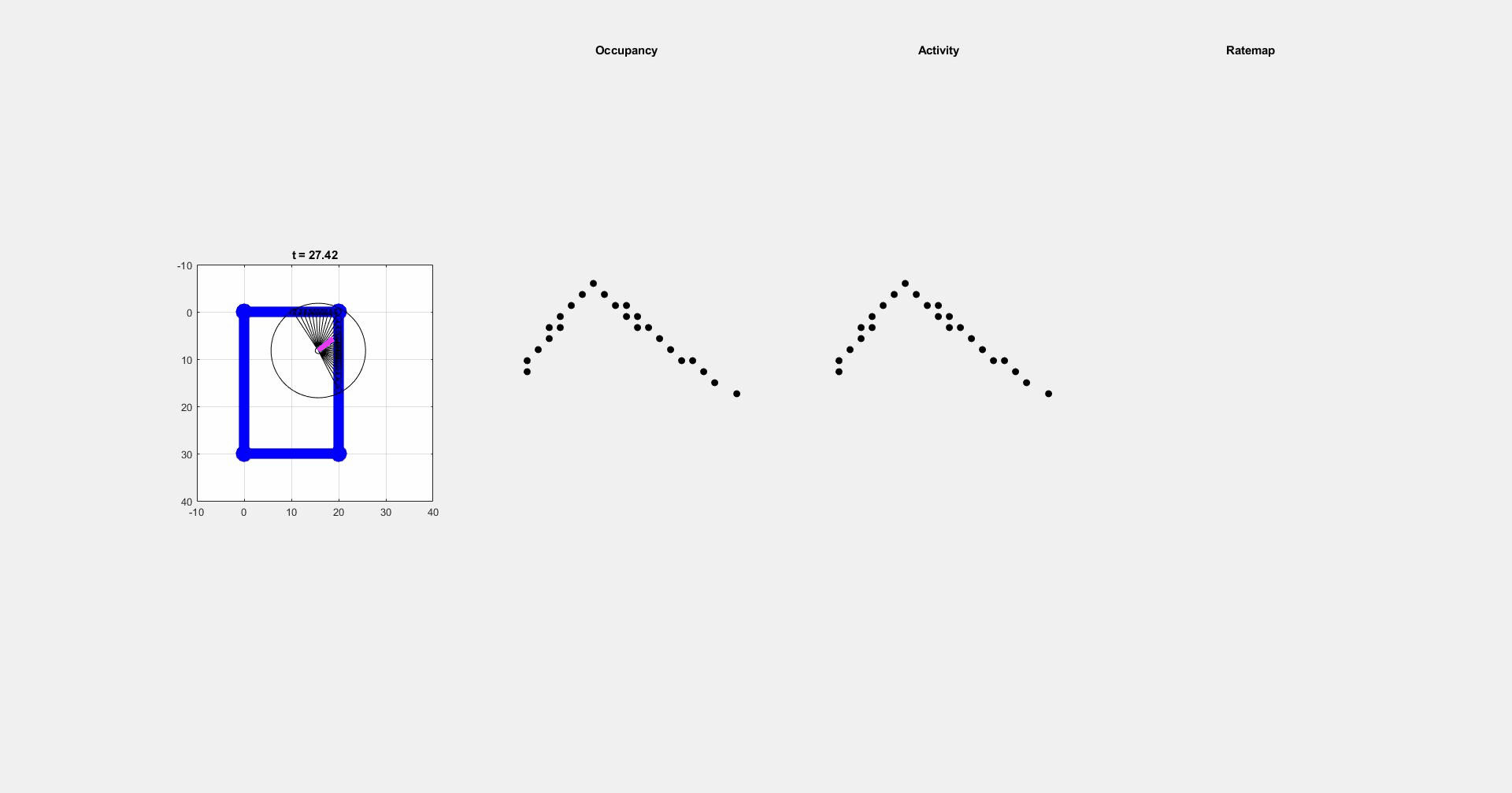
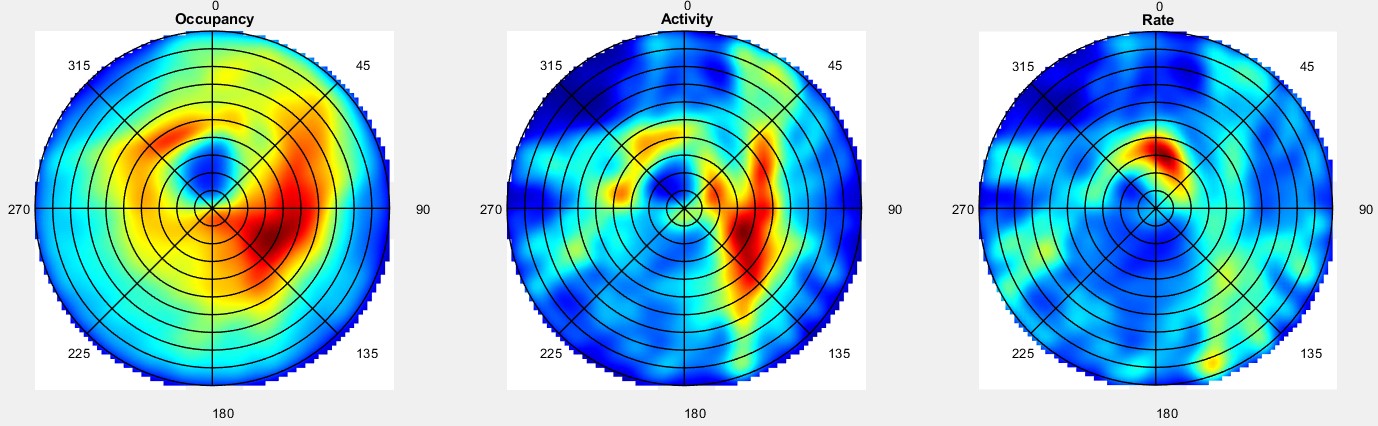
ii. Circuits controlling reorientation
It has been suggested that the strong reliance on geometry during reorientation is due to the stability of these cues in comparison to non-geometric features For example, the appearance of a tree changes from winter to summer, but the shape of the park where the tree is located remains constant. Using stable cues to find shelter, food, or mates is evolutionary advantageous, but the over-reliance on geometry can lead to errors arising from confusion between congruent locations. Therefore, when the directional value and stability of non-geometric cues is learned, animals incorporate non-geometric landmarks in their reorientation strategies to maximize rewards. This raises the following questions: 1) What brain regions send geometric inputs to the hippocampus? 2) What are the brain mechanisms that modulate the use of geometry and features during reorientation? 3) How do brain representations of geometry change over time as animals learn the directional value of other cues?
iii. Applications of reorientation research
Confusional states are particularly prevalent in elderly patients, who often manifest these symptoms during delirium -a state of altered consciousness characterized by spatiotemporal confusion. Delirium is associated with high mortality rates and long-term cognitive decline, it is comorbid with several psychiatric disorders, and is normally associated with hospitalization, infections, and/or metabolic disorders. At present, however, understanding of the brain mechanisms that trigger delirium have been scarce because there are no animal models to assess the time course, cellular manifestations, and/or neurological changes associated with this transient state. Our lab is currently using neuroinflammatory insults to generate states of transient disorientation to evaluate their long-lasting consequences on cognition as well as neurological damage.